38 6.6 Archaea
Learning Objectives
- Describe the unique features of each category of Archaea
- Explain why archaea might not be associated with human microbiomes or pathology
- Give common examples of archaea commonly associated with unique environmental habitats
Like organisms in the domain Bacteria, organisms of the domain Archaea are all unicellular organisms. However, archaea differ structurally from bacteria in several significant ways, as discussed in Unique Characteristics of Prokaryotic Cells. To summarize:
- The archaeal cell membrane is composed of ether linkages with branched isoprene chains (as opposed to the bacterial cell membrane, which has ester linkages with unbranched fatty acids).
- Archaeal cell walls lack peptidoglycan, but some contain a structurally similar substance called pseudopeptidoglycan or pseudomurein.
- The genomes of Archaea are larger and more complex than those of bacteria.
Domain Archaea is as diverse as domain Bacteria, and its representatives can be found in any habitat. Some archaea are mesophiles, and many are extremophiles, preferring extreme hot or cold, extreme salinity, or other conditions that are hostile to most other forms of life on earth. Their metabolism is adapted to the harsh environments, and they can perform methanogenesis, for example, which bacteria and eukaryotes cannot.
Phylogeny
Between 1990 and 2002, archaea were classified into one of two phyla: Euryarchaeota and Crenarchaeota. Today, with advances in technology and analytics, the domain includes at least 4 major supergroups: Euryarchaeota, TACK, Asgard and DPANN (Figure 6.47).[1]
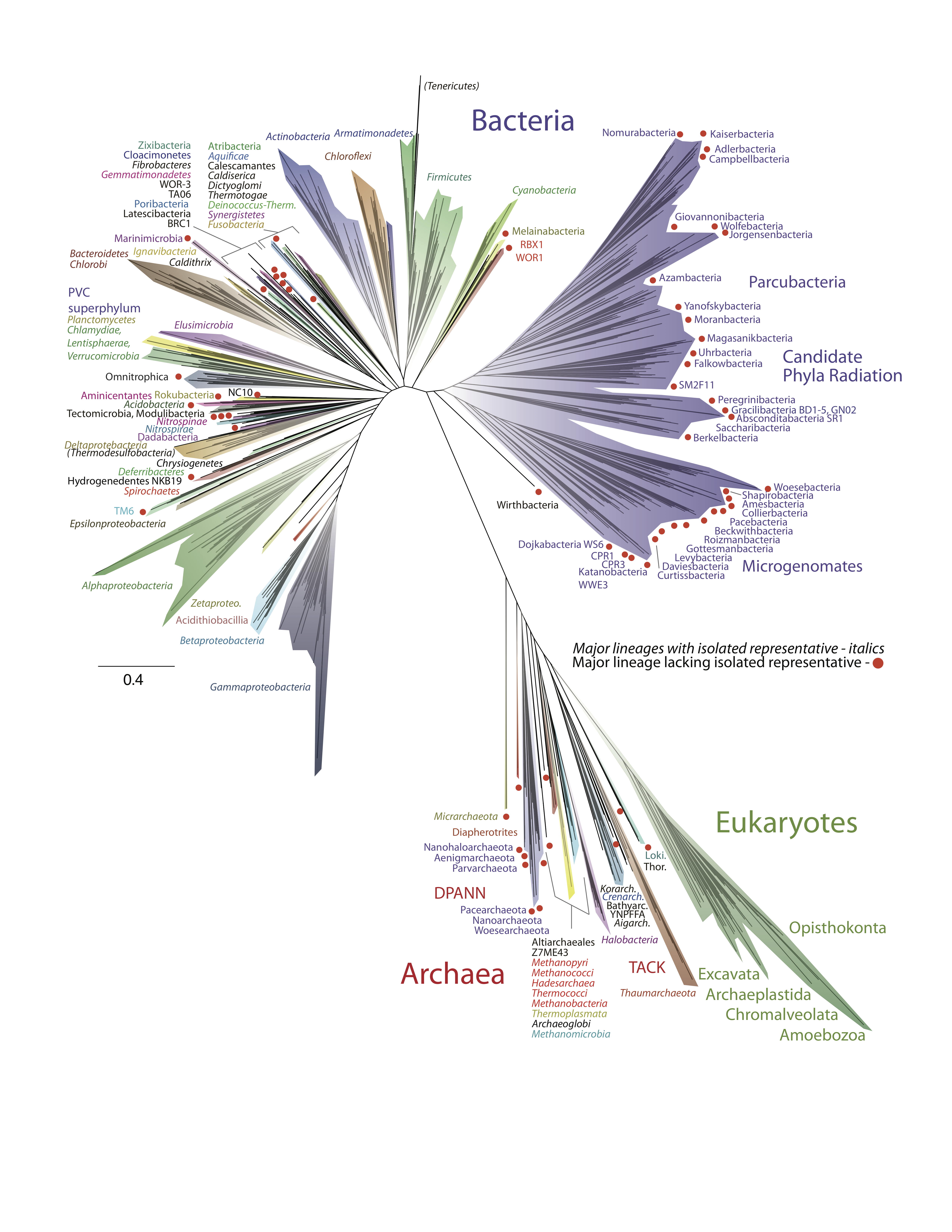
As far as we know, archaea are not associated with infectious diseases in humans, animals, plants, or microorganisms. However, many play important roles in the environment and may thus have an indirect impact on human health.
Metabolism and Physiology
Archaea exhibit a variety of chemical reactions in their metabolism and use many sources of energy. As a result of this and their widespread distribution, archaea play critical roles in cycling of elements in the biosphere. Some archaea are lithotrophs, obtaining energy from inorganic compounds such as elemental sulphur, H2S or ammonia. Lithotrophs are also autotrophic, using CO2 in the atmosphere as a source of carbon, “fixing” the carbon into biomass.
Metabolism
Some archaea are methanogens. These organisms are unique in that they can reduce carbon dioxide in the presence of hydrogen, producing methane. They can live in the most extreme environments and can reproduce at temperatures varying from below freezing to boiling. Methanogens have been found in hot springs as well as deep under ice in Greenland. Some scientists have even hypothesized that methanogens may inhabit the planet Mars because the mixture of gases produced by methanogens resembles the makeup of the Martian atmosphere.[2]
Methanogens are thought to contribute to the formation of anoxic sediments by producing hydrogen sulphide, making “marsh gas.” They also produce gases in ruminants and humans. Some genera of methanogens, notably Methanosarcina, can grow and produce methane in the presence of oxygen, although the vast majority are strict anaerobes.
The class Halobacteria (which was named before scientists recognized the distinction between Archaea and Bacteria) includes halophilic (“salt-loving”) archaea. Halobacteria require a very high concentrations of sodium chloride in their aquatic environment. The required concentration is close to saturation, at 36%; such environments include the Dead Sea as well as some salty lakes in Antarctica and south-central Asia. One remarkable feature of these organisms is that they are phototrophic, using sunlight as a source of energy. They are, however, not photosynthetic. These organisms obtain their carbon and the majority of their energy from organic molecules. However, they possess a light-activated proton pump, bacteriorhodopsin, that generates a proton gradient across the plasma membrane. This gradient is a form of potential energy, the proton motive force. The light-absorbing pigment associated with bacteriorhodopsin gives these archaea, and the bodies of water they inhabit, a beautiful red-purple colour (Figure 6.48).
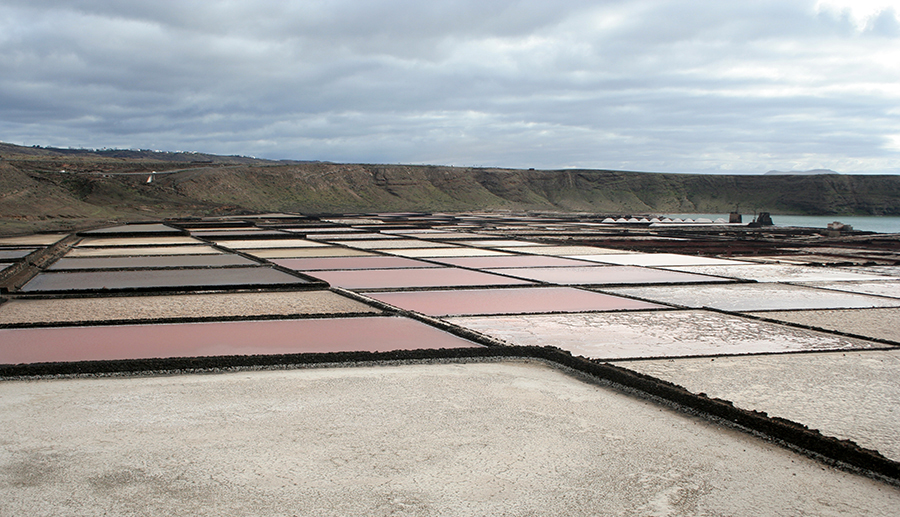
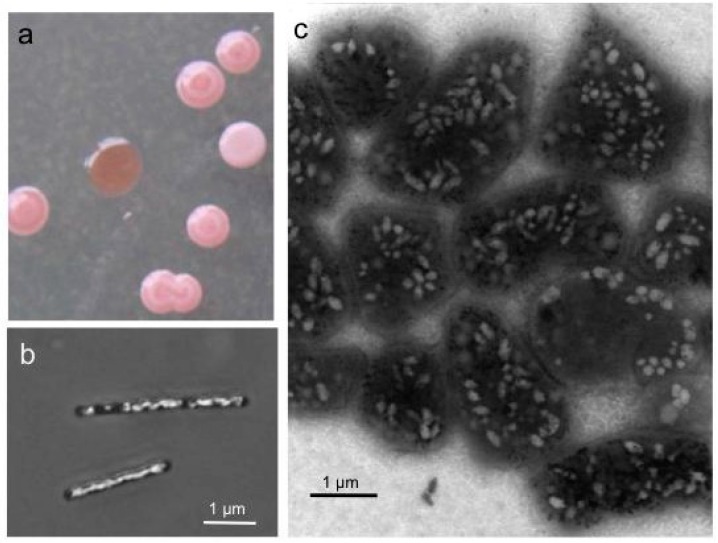
Archaea may be the most abundant organisms in the oceans where they play dominant roles in carbon fixation and ammonia oxidation. Some are hyperthermophiles, with some (notably, the genus Pyrolobus) capable of growth at temperatures up to 113 °C.[5] Hyperthermophilic archaea are essential to deep sea vent ecosystems (Figure 6.50). They form the base of the unique deep sea vent food chain, using the H2S from the vents as their energy source and fixing CO2 .
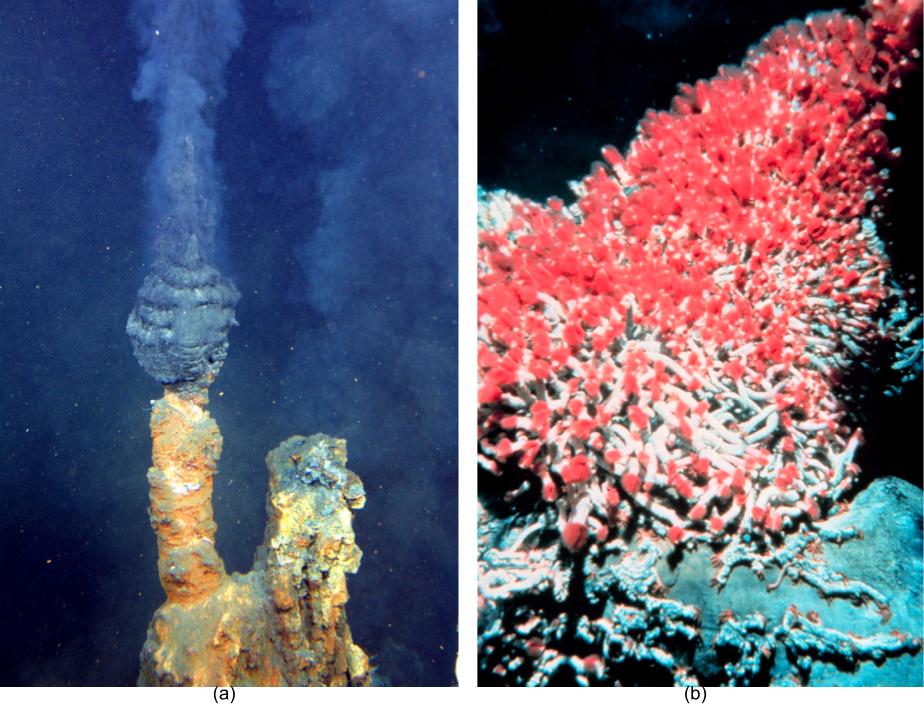
Species in the genus Thermoproteus are strict anaerobes, using sulphur or sulphate as terminal electron acceptors. They are flagellated and with a monolayer plasma membrane and are hyperthermophiles, with an optimal growth temperature of ~85 °C. Thermoproteus species may grow lithotrophically, using hydrogen as an energy source, or they may grow as heterotrophs.
Archaea in the genus Sulfolobus (Figure 6.51) are thermophilic, preferring temperatures around 70–80°C and acidophilic, prefer a pH of 2–3.[6] Sulfolobus can live in aerobic or anaerobic environments. In the presence of oxygen, Sulfolobus spp. use metabolic processes similar to those of heterotrophs. In anaerobic environments, they oxidize sulphur to produce sulphuric acid, which is stored in granules. Sulfolobus spp. are used in biotechnology for the production of thermostable and acid-resistant proteins called affitins.[7] Affitins can bind and neutralize various antigens (molecules found in toxins or infectious agents that provoke an immune response from the body).
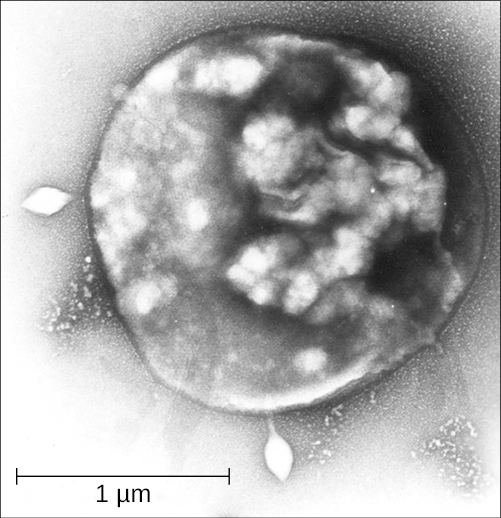
Some other acidophilic archaea obtain their energy from reduced iron. In abandoned mines coal mines, the combination of the metabolic reactions of these and sulphur-oxidizing microbes, plus oxidation from exposure to air, leads to extreme acidification of the water in these mines. The result, acid mine drainage, causes severe ecological damage when gets into the surrounding environment: streams become highly acidic and the low pH causes dissolved minerals to precipitate, thus killing the life forms in those waters (Figure 6.52).
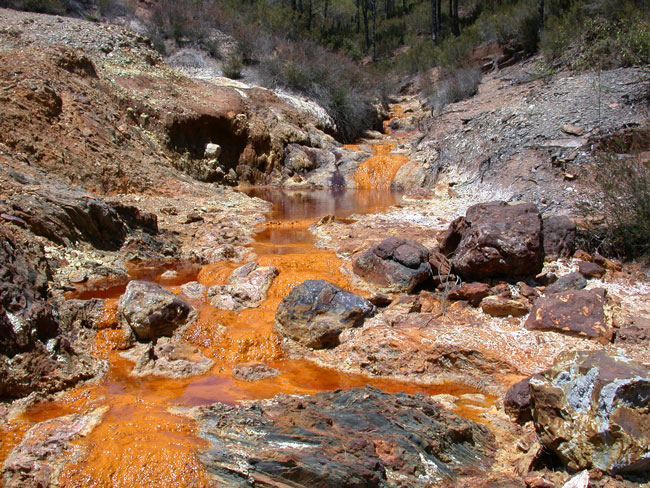
- Describe where Halobacteria live and their metabolism
- Describe the role of hyperthermophilic archaea in deep sea vent food chains
- How do Sulfolobus and other acidophilic archaea give rise to acid mine tailings?
Cell Structure
Until the phylogenetic work of Carl Woese led to the identification of this third domain, archaea were thought to be bacteria. The share many similarities with bacteria: they lack a nucleus or other membrane-bound organelles, putting them into the category of “prokaryote”, most are unicellular, they have 70S sized ribosomes, are typically a few micrometers in size, and reproduce asexually only. They are known to have many of the same structures that bacteria can have, such as plasmids, inclusions, flagella, and pili. Capsules and slime layers have been found but appear to be rare in archaea.
There are, however, many unique features, and many other features that are shared between archaea and eukaryotes. This latter observation, which is reflected in the 2016 tree of life by Hug et al, and more recent ones, supports the proposal that the host for the first endosymbiotic event during eukaryogenesis, was an archaeaon.
Plasma membrane
As described previously, archaeal membrane lipids are ether-linked, as opposed to the ester-linked lipids found in bacteria and eukaryotes. The ether-linkage provides more chemical stability to the membrane. In addition, instead of the unbranched fatty acids characteristics of bacterial and eukaryotic membrane lipids, isoprenoid chains are found in archaea. These isoprenoid chains can have branching side chains and the lipids can exist as monolayers, where the isoprene chains of one phospholipid connect with the isoprene chains of a phospholipid on the opposite side of the membrane. Bacteria and eukaryotes only have lipid bilayers, where the two sides of the membrane remain separated (Figure 6.53).
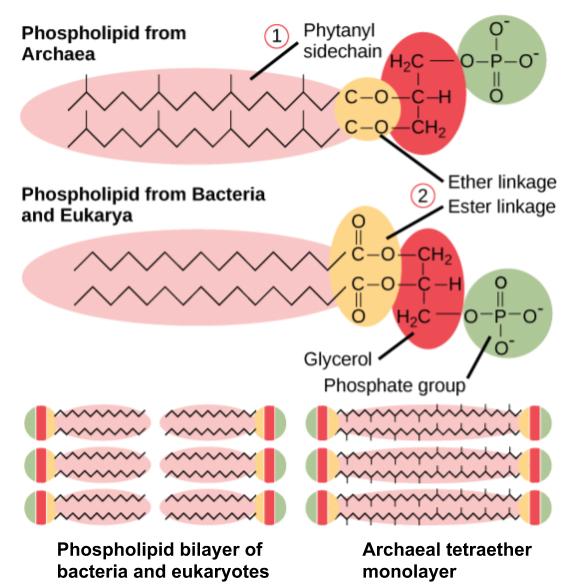
Cell Wall
Like bacteria, the archaeal cell wall is a semi-rigid structure designed to provide protection to the cell from the environment and from the internal cellular pressure. While the cell walls of bacteria typically contain peptidoglycan, that particular chemical is lacking in archaea. Instead, archaea display a wide variety of cell wall types, adapted for the environment of the organism. Some archaea lack a cell wall altogether.
While it is not universal, a large number of Archaea have a proteinaceous S-layer that is considered to be part of the cell wall itself (unlike in bacteria, where an S-layer is a structure in addition to the cell wall). For some archaea the S-layer is the only cell wall component, while in others it is joined by additional ingredients (Figure 6.54). The archaeal S-layer can be made of either protein or glycoprotein, often anchored into the plasma membrane of the cell. The proteins form a two-dimensional crystalline array with a smooth outer surface. A few S-layers are composed of two different S-layer proteins.
While archaea lack peptidoglycan, a few contain a substance with a similar chemical structure, known as pseudomurein. Instead of NAM, it contains N-acetylalosaminuronic acid (NAT) linked to NAG, with peptide interbridges to increase strength.
Methanochondroitin is a cell wall polymer found in some archaeal cells, similar in composition to the connective tissue component chondroitin, found in vertebrates.
Some archaea have a protein sheath composed of a lattice structure similar to an S-layer. These cells are often found in filamentous chains, however, and the protein sheath encloses the entire chain, as opposed to individual cells.
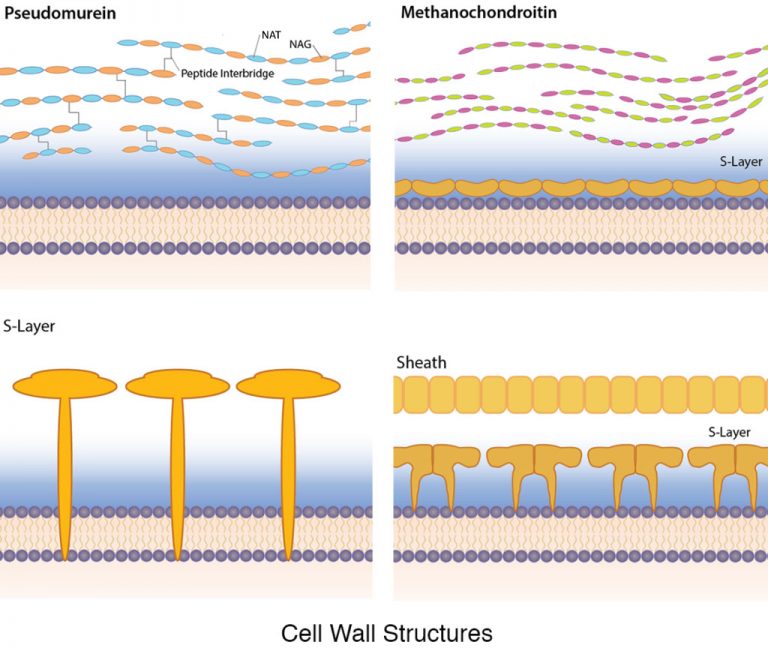
Molecular Biology
Archaea usually have a single circular chromosome, the size of which may be as great as 5,751,492 base pairs in Methanosarcina acetivorans, the largest known archaean genome. One-tenth of this size is the tiny 490,885 base-pair genome of Nanoarchaeum equitans, the smallest archaean genome known. It is estimated to contain only 537 protein-encoding genes. Smaller independent pieces of DNA, called plasmids, are also found in archaea. Plasmids may be transferred between cells by physical contact, in a process that may be similar to bacterial conjugation.
Archaea can be infected by double-stranded DNA viruses that are unrelated to any other form of virus and have a variety of unusual shapes, including bottles, hooked rods, or teardrops. These viruses have been studied in most detail in thermophilics, particularly the orders Sulfolobales (see Figure 6.51) and Thermoproteales. Two groups of single-stranded DNA viruses that infect archaea have been recently isolated. One group is exemplified by the Halorubrum pleomorphic virus 1 (“Pleolipoviridae”) infecting halophilic archaea and the other one by the Aeropyrum coil-shaped virus (“Spiraviridae”) infecting a hyperthermophilic (optimal growth at 90-95°C) host. Notably, the latter virus has the largest currently reported ssDNA genome. Defences against these viruses may involve RNA interference from repetitive DNA sequences that are related to the genes of the viruses.
Archaea are genetically distinct from bacteria and eukaryotes, with up to 15% of the proteins encoded by any one archaeal genome being unique to the domain, although most of these unique genes have no known function. Of the remainder of the unique proteins that have an identified function, most are involved in methanogenesis. The proteins that archaea, bacteria, and eukaryotes share form a common core of cell function, relating mostly to transcription, translation, and nucleotide metabolism. Other characteristic archaean features are the organization of genes of related function—such as enzymes that catalyze steps in the same metabolic pathway into novel operons, and large differences in tRNA genes and their aminoacyl tRNA synthetases.
Transcription and translation in archaea resemble these processes in eukaryotes more than in bacteria, with the archaeal RNA polymerase and ribosomes being very close to their equivalents in eukaryotes. Although archaea only have one type of RNA polymerase, its structure and function in transcription seems to be close to that of the eukaryotic RNA polymerase II, with similar protein assemblies (the general transcription factors) directing the binding of the RNA polymerase to a gene’s promoter. However, other archaean transcription factors are closer to those found in bacteria. Post-transcriptional modification is simpler than in eukaryotes, since most archaean genes lack introns, although there are many introns in their transfer RNA and ribosomal RNA genes, and introns may occur in a few protein-encoding genes.
MICRO CONNECTIONS: Finding a Link Between Archaea and Disease
Archaea are not known to cause any disease in humans, animals, plants, bacteria, or in other archaea. Although this makes sense for the extremophiles, not all archaea live in extreme environments. Many genera and species of Archaea are mesophiles, so they can live in human and animal microbiomes, although they rarely do. As we have learned, some methanogens exist in the human gastrointestinal tract. Yet we have no reliable evidence pointing to any archaean as the causative agent of any human disease.
Still, scientists have attempted to find links between human disease and archaea. For example, in 2004, Lepp et al. presented evidence that an archaean called Methanobrevibacter oralis inhabits the gums of patients with periodontal disease. The authors suggested that the activity of these methanogens causes the disease.[8] However, it was subsequently shown that there was no causal relationship between M. oralis and periodontitis. It seems more likely that periodontal disease causes an enlargement of anaerobic regions in the mouth that are subsequently populated by M. oralis.[9]
There remains no good answer as to why archaea do not seem to be pathogenic, but scientists continue to speculate and hope to find the answer.
Additional Resources
Key Takeaways
- Archaea are unicellular, prokaryotic microorganisms that differ from bacteria in their genetics, biochemistry, and ecology.
- Some archaea are extremophiles, living in environments with extremely high or low temperatures, or extreme salinity.
- Only archaea are known to produce methane. Methane-producing archaea are called methanogens.
- Halophilic archaea prefer a concentration of salt close to saturation and perform photosynthesis using bacteriorhodopsin.
- Archaeal membrane lipids are ether-linked and may form bilayers or monolayers
- Archaeal cell walls may consist of pseudomurein or proteinaceous S-layers
- Archaea may be infected by viruses.
- Many unique proteins are encoded by archaea, many of these proteins have unknown functions.
- Introns are more rare than eukaryotic species, and additionally unlike eukaryotes the introns usually do not reside in protein coding genes but rather rRNA and tRNA.
- Some archaea, based on fossil evidence, are among the oldest organisms on earth.
- Archaea do not live in great numbers in human microbiomes and are not known to cause disease.
Multiple Choice
Fill in the Blank
Short Answer
- What accounts for the purple colour in salt ponds inhabited by halophilic archaea?
- What evidence supports the hypothesis that some archaea live on Mars?
Critical Thinking
- What is the connection between this methane bog and archaea (credit: Chad Skeers)
- L.A. Hug et al. "A new view of the tree of life". Nat Microbiol. 1 (2016):16048. doi: 10.1038/nmicrobiol.2016.48. ↵
- R.R. Britt “Crater Critters: Where Mars Microbes Might Lurk.” http://www.space.com/1880-crater-critters-mars-microbes-lurk.html. Accessed May 13, 2019. ↵
- H. Vreeland et al. “Fatty acid and DA Analyses of Permian Bacterium Isolated From Ancient Salt Crystals Reveal Differences With Their Modern Relatives.” Extremophiles 10 (2006):71–78. ↵
- F. Pfeifer. "Haloarchaea and the formation of gas vesicles". Life 5 (2015):385–402. doi: 10.3390/life5010385. ↵
- . E. Blochl et al. “Pyrolobus fumani, gen. and sp. nov., represents a novel group of Archaea, extending the upper temperature limit for life to 113°C.” Extremophiles 1 (1997):14–21. ↵
- T.D. Brock et al. “Sulfolobus: A New Genus of Sulfur-Oxidizing Bacteria Living at Low pH and High Temperature.” Archiv für Mikrobiologie 84 no. 1 (1972):54–68. ↵
- S. Pacheco et al. “Affinity Transfer to the Archaeal Extremophilic Sac7d Protein by Insertion of a CDR.” Protein Engineering Design and Selection 27 no. 10 (2014):431-438. ↵
- P.W. Lepp et al. “Methanogenic Archaea and Human Gum Disease.” Proceedings of the National Academies of Science of the United States of America 101 no. 16 (2004):6176–6181. ↵
- R.I. Aminov. “Role of Archaea in Human Disease.” Frontiers in Cellular and Infection Microbiology 3 (2013):42. ↵