15 3.6 Temperature and Microbial Growth
Learning Objectives
- Illustrate and briefly describe minimum, optimum, and maximum temperature requirements for growth
- Identify and describe different categories of microbes with temperature requirements for growth: psychrophile, psychrotrophs, mesophile, thermophile, hyperthermophile
- Give examples of microorganisms in each category of temperature tolerance
When the exploration of Lake Whillans started in Antarctica, researchers did not expect to find much life. Constant subzero temperatures and lack of obvious sources of nutrients did not seem to be conditions that would support a thriving ecosystem. To their surprise, the samples retrieved from the lake showed abundant microbial life. In a different but equally harsh setting, bacteria and archaea grow at the bottom of the ocean around deep sea vents (Figure 3.38), where temperatures can reach 340 °C (700 °F). In fact, some of those bacteria and archaea are the primary producers of the vent ecosystem, providing fixed carbon for the other organisms.
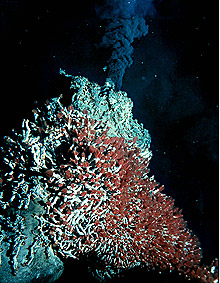
Unlike other environmental conditions such as pH or osmolarity, microbes have no way to regulate their temperature: their internal temperature matches that of their environment. Changes in temperature have the biggest effect on enzymes and their activity, with an optimal temperature that leads to the fastest metabolism and resulting growth rate. Temperatures below optimal will lead to a decrease in enzyme activity and slower metabolism, while higher temperatures can actually denature proteins such as enzymes and carrier proteins, leading to cell death. As a result, microbes have a growth curve in relation to temperature with an optimal temperature at which growth rate peaks, as well as minimum and maximum temperatures where growth continues but is not as robust. For a bacterium, the growth range is typically around 30 degrees (Figure 3.39).
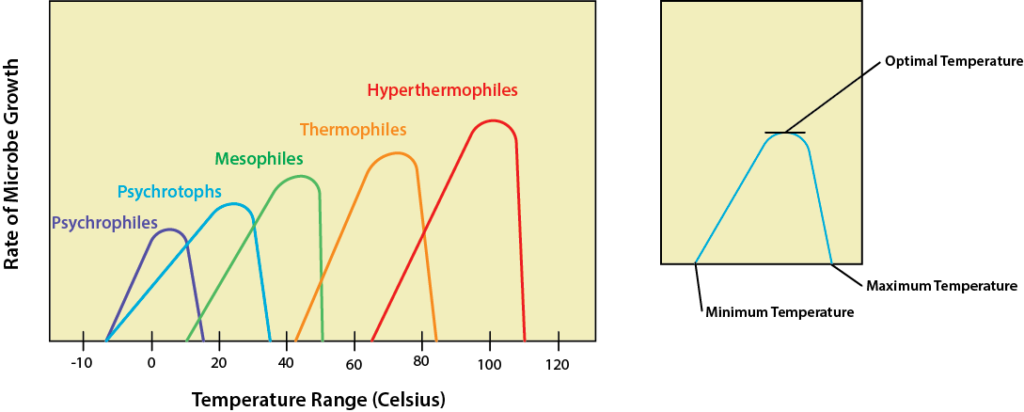
The upward trend of growth rates between psychrophiles and hyperthermophiles is attributed to the relationship between thermal energy and the rate of chemical reactions and has been termed the “Arrhenius effect”. This is a reference to the Arrhenius equation that describes the relationship between temperature and the velocity of a chemical reaction.
Mesophiles
Organisms categorized as mesophiles (“middle loving”) are adapted to moderate temperatures, with optimal growth temperatures ranging from room temperature (about 20 °C) to about 45 °C. As would be expected from the core temperature of the human body, 37 °C (98.6 °F), normal human microbiota and pathogens (e.g., E. coli, Salmonella spp., and Lactobacillus spp.) are mesophiles. The mesophiles occupy the same environments that humans do, in terms of foods that we eat, surfaces that we touch, and water that we swim in and drink.
Psychrophiles
The psychrophiles are the cold lovers, with an optimum of 15oC or lower and a growth range of -20oC to 20oC. Most of these microbes are found in the oceans, where the temperature is often 5oC or colder. They can also be found in the Arctic and the Antarctic, living in ice wherever they can find pockets of liquid water. The organisms retrieved from arctic lakes such as Lake Whillans are considered extreme psychrophiles. Adaptation to the cold required evolution of specific proteins, particularly enzymes, that can still function in low temperatures. These enzymes are more flexible than their mesophilic and thermophilic homologues and they have more accessible catalytic sites to accommodate slower diffusion rates. This increase in flexibility came at a cost, as psychrophilic proteins rapidly denature above their temperature optima. Adaptation to growth at lower temperatures also required modification to the plasma membrane to keep it semifluid. Psychrophiles have an increased amount of unsaturated and shorter-chain fatty acids. Lastly, psychrophiles produce cryoprotectants: special proteins or sugars that prevent the development of damaging ice crystals. Psychrotophs or cold tolerant microbes have a range of 0-35oC, with an optimum of 16oC or higher. They are found in many natural environments in temperate climates and are responsible for the spoilage of refrigerated food. The human pathogen Listeria monocytogenes is an example. It grows in the guts of cattle, can contaminate beef, milk and crops but unlike typical mesophilic human pathogens, it grows at refrigerated temperatures. Food-borne infections result from the consumption of ready-to-eat foods, including lettuce, unpasteurized cheeses and cold-cuts. Because they are active at low temperature, psychrophiles and psychrotrophs are important decomposers in cold climates. and their enzymes are of interest to biotechnology.
CLINICAL FOCUS: Resolution
The presence of Listeria in Jeni’s blood suggests that her symptoms are due to listeriosis, an infection caused by L. monocytogenes. Listeriosis is a serious infection with a 20% mortality rate and is a particular risk to Jeni’s fetus. A sample from the amniotic fluid cultured for the presence of Listeria gave negative results. Because the absence of organisms does not rule out the possibility of infection, a molecular test based on the nucleic acid amplification of the 16S ribosomal RNA of Listeria was performed to confirm that no bacteria crossed the placenta. Fortunately, the results from the molecular test were also negative.
Jeni was admitted to the hospital for treatment and recovery. She received a high dose of two antibiotics intravenously for 2 weeks. The preferred drugs for the treatment of listeriosis are ampicillin or penicillin G with an aminoglycoside antibiotic. Resistance to common antibiotics is still rare in Listeria and antibiotic treatment is usually successful. She was released to home care after a week and fully recovered from her infection.
L. monocytogenes is a gram-positive short rod found in soil, water, and food. It is classified as a psychrotroph and is also halotolerant. Its ability to multiply at refrigeration temperatures (4–10 °C) and its tolerance for high concentrations of salt (up to 10% sodium chloride [NaCl]) make it a frequent source of food poisoning. Because Listeria can infect animals, it often contaminates food such as meat, fish, or dairy products. Contamination of commercial foods can often be traced to persistent biofilms that form on manufacturing equipment that is not sufficiently cleaned.
Listeria infection is relatively common among pregnant women because the elevated levels of progesterone down-regulate the immune system, making them more vulnerable to infection. The pathogen can cross the placenta and infect the fetus, often resulting in miscarriage, stillbirth, or fatal neonatal infection. Pregnant women are thus advised to avoid consumption of soft cheeses, refrigerated cold cuts, smoked seafood, and unpasteurized dairy products. Because Listeria bacteria can easily be confused with diphtheroids, another common group of gram-positive rods, it is important to alert the laboratory when listeriosis is suspected.
Go back to the previous Clinical Focus box.
Thermophiles and Hyperthermophiles
Organisms that grow at optimum temperatures of 50 °C to a maximum of 80 °C are called thermophiles (“heat loving”). They do not multiply at room temperature. Thermophiles are widely distributed in hot springs, geothermal soils, and manmade environments such as garden compost piles where the microbes break down organic waste. Examples of thermophiles include Thermus aquaticus and Geobacillus spp. Higher up on the extreme temperature scale we find the hyperthermophiles, which are characterized by growth ranges from 80 °C to a maximum of 110 °C, with some extreme examples that survive temperatures above 121 °C, the average temperature of an autoclave. The hydrothermal vents at the bottom of the ocean are a prime example of extreme environments, with temperatures reaching an estimated 340 °C (Figure 3.38). Microbes isolated from the vents achieve optimal growth at temperatures higher than 100 °C. Noteworthy examples are Pyrobolus and Pyrodictium, archaea that grow at 105 °C and survive autoclaving. Both the thermophiles and the hyperthermophiles require specialized heat-stable enzymes that are resistant to denaturation and unfolding. In contrast to their psychrophilic homologues, they are more tightly folded, making them less flexible with a less accessible catalytic site. In addition, these organisms express protective chaperone proteins to help with protein folding and to help maintain their native structure. The enzymes of these organisms are also of interest to biotechnology. It was the discovery of Thermus aquaticus that led biochemist Kary Mullis to invent the revolutionary technique of PCR (Polymerase Chain Reaction), using the organism’s heat-active DNA polymerase (the Taq polymerase). This technique is used in every field of biology and, in combination with sequencing advances and the development of metagenomics, has revolutionized the field of microbiology. Dr. Mullis was awarded the Nobel Prize in Chemistry in 1993.
Adaptation to higher growth temperatures also required adaptations to maintain the semifluid consistency of the plasma membrane. The membrane lipids of these organisms contain a high content of saturated fatty acids. Due to their linear structures, saturated lipids pack more tightly, giving a more organized membrane and increasing its melting temperature. In addition, the ether linkage of the thermophilic and hyperthermophilic archaea is more thermostable than the ester linkage of phospholipids. The high thermal stability of the hyperthermophilic archaeal membranes is also due to their tetraether monolayer structure, as the inner and outer layers of a membrane bilayer will separate under very high temperatures.
Adaptation to Temperature Fluctuations
The ability to adapt to fluctuations in temperature allows organisms to survive, if not grow, at temperatures at or near the minima and maxima. Most organisms can alter the types of lipids they are synthesizing in response to such fluctuations. In addition, the induction of heat shock, or cold shock proteins are global stress responses that involve the expression of chaperone proteins that may help fold unfolded proteins or may form protective shells around proteins to prevent their denaturation. It is important to remember however, that this ability does not alter the organism’s optimal growth temperature: so the fact that E. coli might survive in an undercooked hamburger does not make it a thermophile or hyperthermophile!
Practical Applications
Some of the practical applications of the destructive effects of heat on microbes are sterilization by steam, pasteurization, and incineration of inoculating loops. While refrigeration and freezing are used for food preservation, freezing at−80 °C, or even lower with liquid nitrogen, is used for long-term preservation of bacterial and archaeal cultures. The damaging effects of ice crystal formation can be avoided by mixing liquid suspensions of cells with sterile solutions of the cryoprotectant glycerol. Cultures can withstand freeze drying (lyophilization) and then be stored as powders in sealed ampules to be reconstituted with broth when needed.
Life in extreme environments raises fascinating questions about the adaptation of macromolecules and metabolic processes. Astrobiology is a relatively new discipline that involves multidisciplinary research into the origin, evolution and distribution of life in the universe. Studies of the psychrophiles and (hyper)thermophiles can inform our understanding of the evolution of life on Earth (and possibly elsewhere) during the young planet’s warmer days, and the possible existence of life in the generally colder environments in space, and in particular, on Mars.
Visit NASA’s astrobiology website to learn more about this discipline, and learn how studies of extremophilic cyanobacteria can provide information on the possibility of microbial life on Mars
- What temperature requirements do most bacterial human pathogens have?
- What DNA adaptation do thermophiles exhibit?
Key Takeaways
- Microorganisms thrive at a wide range of temperatures; they have colonized different natural environments and have adapted to extreme temperatures. Both extreme cold and hot temperatures require evolutionary adjustments to macromolecules and biological processes.
- Psychrophiles grow best in the temperature range of 0–15 °C whereas psychrotrophs thrive between 4°C and 25 °C.
- Mesophiles grow best at moderate temperatures in the range of 20 °C to about 45 °C. Pathogens are usually mesophiles.
- Thermophiles and hyperthemophiles are adapted to life at temperatures above 50 °C.
- Adaptations to cold and hot temperatures require changes in the composition of membrane lipids and proteins.
Multiple Choice
Drag and Drop
Short Answer
- How are hyperthermophile’s proteins adapted to the high temperatures of their environment?
- Why would NASA be funding microbiology research in Antarctica?
Critical Thinking
- The bacterium that causes Hansen’s disease (leprosy), Mycobacterium leprae, infects mostly the extremities of the body: hands, feet, and nose. Can you make an educated guess as to its optimum temperature of growth?
- Refer to Figure 9.39. Some hyperthermophiles can survive autoclaving temperatures. Are they a concern in health care?