14 3.5 The Effects of pH on Microbial Growth
Learning Objectives
- Illustrate and briefly describe minimum, optimum, and maximum pH requirements for growth
- Identify and describe the different categories of microbes with pH requirements for growth: acidophiles, neutrophiles, and alkaliphiles
- Give examples of microorganisms for each category of pH requirement
Yogurt, pickles, sauerkraut, and lime-seasoned dishes all owe their tangy taste to a high acid content (Figure 3.34). Recall that acidity is a function of the concentration of hydrogen ions [H+] and is measured as pH. Environments with pH values below 7.0 are acidic, with a high concentration of H+ ions; those with pH values above 7.0 are considered basic. Extreme pH affects the structure of all macromolecules. The hydrogen bonds holding together strands of DNA break up at high pH. Lipids are hydrolyzed by an extremely basic pH. The proton motive force responsible for production of ATP in cellular respiration depends on the concentration gradient of H+ across the plasma membrane (see Cellular Respiration). If H+ ions are neutralized by hydroxide ions, the concentration gradient collapses and impairs energy production. But the component most sensitive to pH in the cell is its workhorse, the protein. Moderate changes in pH modify the ionization of amino-acid functional groups and disrupt hydrogen bonding, which, in turn, promotes changes in the folding of the molecule, promoting denaturation and destroying activity.

The optimum growth pH is the most favourable pH for the growth of an organism. The lowest pH value that an organism can tolerate is called the minimum growth pH and the highest pH is the maximum growth pH (Figure 3.35). These values can cover a wide range, which is important for the preservation of food and to microorganisms’ survival in the stomach. For example, the optimum growth pH of Salmonella spp. is 7.0–7.5, but the minimum growth pH is closer to 4.2.
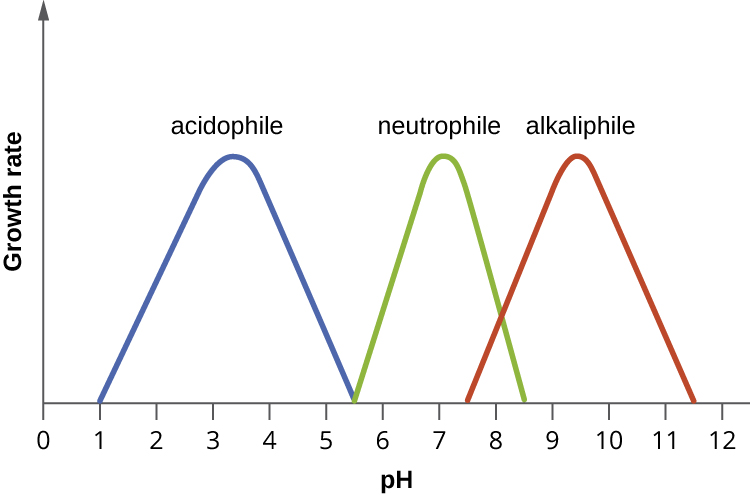
Neutrophiles
Most bacteria are neutrophiles, meaning they grow optimally at a pH within one or two pH units of the neutral pH of 7, between 5 and 8 (see Figure 3.35). Most familiar bacteria, like Escherichia coli, staphylococci, and Salmonella spp. are neutrophiles and do not fare well in the acidic pH of the stomach. However, there are pathogenic strains of E. coli, S. typhi, and other species of intestinal pathogens that are much more resistant to stomach acid. In comparison, fungi thrive at slightly acidic pH values of 5.0–6.0.
Acidophiles
Microorganisms that grow optimally at a pH less than 5 are called acidophiles. For example, the sulphur-oxidizing Sulfolobus spp. isolated from sulphur mud fields and hot springs in Yellowstone National Park are extreme acidophiles. These archaea survive at pH values of 2.5–3.5. Species of the archaean genus Ferroplasma live in acid mine drainage at pH values of 0–2.9 (Figure 3.36). Lactobacillus bacteria, which are an important part of the normal microbiota of the vagina, can tolerate acidic environments at pH values 3.5–6.8 and also contribute to the acidity of the vagina (pH of 4, except at the onset of menstruation) through their metabolic production of lactic acid. The vagina’s acidity plays an important role in inhibiting other microbes that are less tolerant of acidity. Acidophilic microorganisms display a number of adaptations to survive in strong acidic environments. While the membrane is slightly leaky to protons, the cytoplasmic pH of most acidophiles is generally only slightly acidic. One of the major reasons for this is their ability to actively transport of H+ ions out of the cell. In addition, cytoplasmic proteins have evolved to function better at a slightly acidic pH with increased negative surface charges compared to their neutrophilic homologues. The ether linkage of the archaeal membrane lipids is more acid stable than the typical ester linked phospholipids, but in addition, acidophilic archaea typically possess tetraether membrane lipids. The resulting monolayer structure makes their membranes a much better barrier to proton leakage in extremely low pH environments. And since these organisms may also be adapted to growing at high temperatures, the membranes also maintain their semi-fluid consistency. While the cytoplasmic proteins of acidophiles have relatively normal pH optima, those that are secreted have acidic pH optima compared to their neutrophile homologues. The gene sequences for acidophilic secreted proteins have evolved to give secondary, tertiary and quaternary structures that are resistant to the protonating effects of the acidic environment. These proteins are of great interest for their possible biotechnological applications.
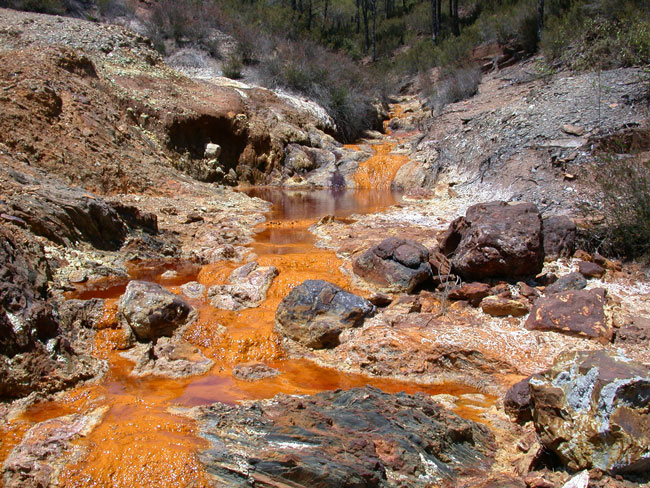
Alkaliphiles
At the other end of the spectrum are alkaliphiles, microorganisms that have pH optima between 8.0 and 11. Vibrio cholerae, the pathogenic agent of cholera, grows best at the slightly basic pH of 8.0; it can survive pH values of 11.0 but is inactivated by the acid of the stomach. When it comes to survival at high pH, the bright pink halophilic archaeon Natronobacterium, found in the soda lakes of the African Rift Valley, may hold the record at a pH of 10.5 (Fig. 3.37). Extreme alkaliphiles have adapted to their harsh environment through various evolutionary modifications. Alkaliphilic archaea have diether lipid membranes. The ether linkage is more resistant to chemical or thermal degradation compared to the ester-linked phospholipids. Given the paucity of protons in alkaline environments, maintaining a proton motive force is probably the most pressing challenge for alkaliphiles. One of the adaptations of alkaliphilic halophilic bacteria and archaea in soda lakes and other highly salty environments is the evolution of coupled transporters and flagella that exploit sodium motive force, thus conserving the PMF for oxidative and photophosphorylation by the ATP synthase. The cell surface of alkaliphiles has a high concentration of acidic (i.e. negatively charged) molecules and it has been suggested this acts as a “proton sponge”, allowing a more rapid lateral diffusion of protons from the ETS, to the ATP synthase, compared to the rate of diffusion into the surrounding waters[1] Finally, alkaliphiles may use Na+/H+ antiport to create a sodium motive force. For example, the alkaliphile Bacillus firmus derives the energy for transport reactions and motility from SMF rather than a proton motive force. As with the acidophiles, the genes for secreted proteins of alkaliphiles have evolved to give enzymes that resist deprotonation/denaturation and chemical degradation at the high pH of their environment. These enzymes are also of interest to biotechnology companies. In fact, laundry detergents, which are alkaline in nature, contain alkaliphilic lipases and proteases to improve their stain-removing abilities.
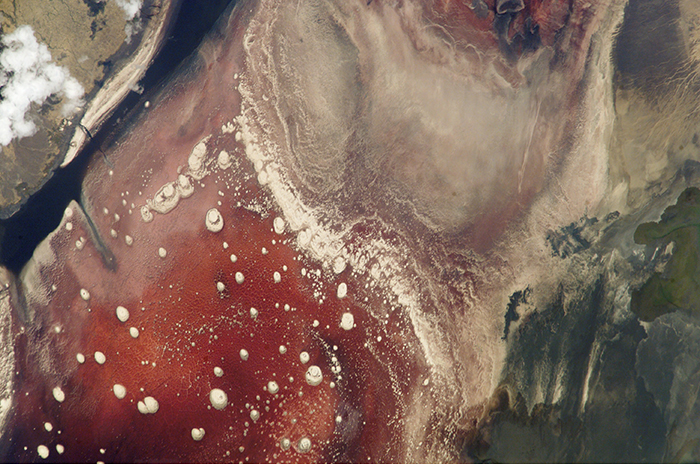
Adapting to Changes in Environmental pH
Outside of the lab, the physicochemical conditions faced by microbes may fluctuate rapidly. This is also true of host-associated microbes. The bacteria and archaea of the developing gut microbiota, as well as gastrointestinal pathogens such as the various pathogenic strains of E. coli, must be able to survive exposure to the acid pH of the stomach in order to colonize intestinal tract. These organisms therefore require the ability to adapt to a sudden and temporary drop in pH. One major strategy such organisms use is a global stress response system known as the “acid shock” response. This response results in acid tolerance and involves the induction (turning on) of a suite of genes, and the repression of others to essentially reprogram the cell. Among the molecules that are expressed as a result of acid stress are chaperones. These function to help fold, or refold, unfolded proteins. This response helps neutrophiles survive exposure to acid pH but below the minimum, proteins are irreversibly denatured and the cell dies. This has been exploited as a means of food preservation (Figure 3.34), for example in pickling (vinegar is dilute acetic acid).
MICRO CONNECTIONS: Survival at the Low pH of the Stomach
Peptic ulcers (or stomach ulcers) are painful sores on the stomach lining. Until the 1980s, they were believed to be caused by spicy foods, stress, or a combination of both. Patients were typically advised to eat bland foods, take anti-acid medications, and avoid stress. These remedies were not particularly effective, and the condition often recurred. This all changed dramatically when the real cause of most peptic ulcers was discovered to be a slim, corkscrew-shaped bacterium, Helicobacter pylori. This organism was identified and isolated by Barry Marshall and Robin Warren, whose discovery earned them the Nobel Prize in Medicine in 2005.
The ability of H. pylori to survive the low pH of the stomach would seem to suggest that it is an extreme acidophile. As it turns out, this is not the case. In fact, H. pylori is a neutrophile. So, how does it survive in the stomach? Remarkably, H. pylori creates a microenvironment in which the pH is nearly neutral. It achieves this by producing large amounts of the enzyme urease, which breaks down urea to form NH4+ and CO2. The ammonium ion raises the pH of the immediate environment.
This metabolic capability of H. pylori is the basis of an accurate, noninvasive test for infection. The patient is given a solution of urea containing radioactively labeled carbon atoms. If H. pylori is present in the stomach, it will rapidly break down the urea, producing radioactive CO2 that can be detected in the patient’s breath. Because peptic ulcers may lead to gastric cancer, patients who are determined to have H. pylori infections are treated with antibiotics.
- What effect do extremes of pH have on proteins?
- What pH-adaptive type of bacteria would most human pathogens be?
Key Takeaways
- Bacteria are generally neutrophiles. They grow best at neutral pH close to 7.0.
- Acidophiles grow optimally at a pH near 3.0. Alkaliphiles are organisms that grow optimally between a pH of 8 and 10.5. Extreme acidophiles and alkaliphiles grow slowly or not at all near neutral pH.
- Microorganisms grow best at their optimum growth pH. Growth occurs slowly or not at all below the minimum growth pH and above the maximum growth pH.
Multiple Choice
Fill in the Blank
Short Answer
- Which macromolecule in the cell is most sensitive to changes in pH?
- Which metabolic process in the bacterial cell is particularly challenging at high pH?
Critical Thinking
- People who use proton pumps inhibitors or antacids are more prone to infections of the gastrointestinal tract. Can you explain the observation in light of what you have learned?
- Mulkidjanian AY, Heberle J, Cherepanov DA. 2006. Protons @ interfaces: implications for biological energy conversion. Biochim Biophys Acta. 1757(8):913-30. ↵