Introduction
lgeorge2
Welcome to the fascinating world of Astrodynamics! In this course, you will learn about orbital mechanics, maneuvers, and their basic applications. Have you ever wondered why we launch spacecraft from specific locations and during certain time windows? What is a geosynchronous orbit, and what is needed to get satellites there? How do spacecraft get to Mars and other interplanetary destinations? In this book, you will find the answers to these questions and others. You will learn about satellite orbits and why specific ones are chosen as well as how to change satellite orbits. It should be a great learning experience and will be helpful in your chosen career field.
For an introduction to the author and additional information about orbital mechanics, see Video 1. For information about the primary editor, see Video 2.
Video 1: Instructor information
Video 2: Editor Information
Expected Outcomes:
- Understand the basic laws of motion and their effect on spaceflight dynamics
- Distinguish between different types of orbits
- Use Kepler’s equations to predict a satellite’s future location
- Understand the causes and effects of orbit perturbations
- Design maneuvers for transferring spacecraft between orbits
- Understand the dynamics of spacecraft rendezvous
- Perform preliminary design of interplanetary missions
- Understand when, where, and how to launch spacecraft into orbit
- Know the fundamentals of advanced orbital mechanics techniques
This book is divided into roughly twelve parts, the last two of which are optional for an introductory course.
- Orbital Basics
- Orbit Geometry
- The Classical Orbital Elements
- Preliminary Orbit Determination
- Launch Windows and Time
- Kepler’s Time of Flight and Prediction Problem
- Orbital Maneuvering
- Rendezvous
- Ground tracks
- Orbital Perturbations
- Interplanetary Travel
- Launching into Space
- Intro to Advanced Orbital Mechanics
See the Schedule for a detailed list of topics and schedule by lesson.
These topics cover enough fundamental information to give you insight into orbital mechanics, regardless of whether you are a student, professional, or educator. It is intended for people who have a basic background in mathematics and physics; however, an in-depth understanding of those topics is not required.
At this time, I would like you to pause and think about the reasons why we want to go into space. What types of space missions do we have currently? Some people argue that space systems are a waste of time, money, and valuable resources. What would you say to counter that claim?
OVERVIEW
Space systems give us a global perspective and serve as the ultimate high ground (Sellers and Astore). What types of orbits do we use for space missions? You probably know we use weather satellites and other earth observing missions from relatively low orbits, lower than about 500 km (310 miles). But do you know how these ideas got started?
Manned balloon flights were recorded as early as the 1700s in France. By the 1790s, the French military had formed balloon units for observation purposes. The Union army used tethered, gas-filled balloons to observe enemy troops and to direct artillery fire during the Civil War (Figure 1). The Confederate soldiers would try to bring them down, but the balloons would float just beyond the range of enemy guns (Military History Now, 2015). Today, we regularly use Google Maps and other satellite-based imagery to get as global or close a perspective as we need.
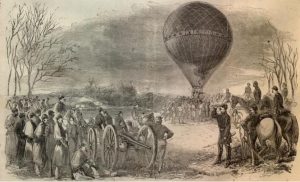
An advantage of space systems is that we can see a LONG WAY, unobscured by Earth’s atmosphere. The James Webb Space Telescope (JWST) took this photo of the Carina Nebula, one of our galaxy’s largest star-forming regions (Figure 2). It is at a distance of 7500 light years and has a diameter of over 200 light years. The JWST was launched in 2021 into Earth-Sun Lagrange point 2 (L2). It orbits the Sun at about 1.5 million kilometers (km), or 932,000 miles, away from the Earth. Lagrange points in space are locations at which three bodies can orbit each other yet stay in the same position relative to each other (Webb/NASA). These are very stable orbits, and you will find out more about why L2 is a fitting orbit for the JWST later this course (NASA, 2023). The telescope is the largest, most powerful, and most complex one to date. It is an infrared observatory that has longer wavelength coverage and better sensitivity than previous telescopes.
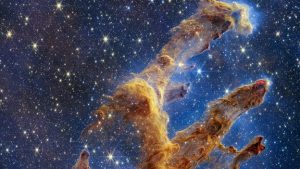
Its predecessors, the Hubble Space Telescope (HST) and the Kepler Space Telescope (KST), were the pathfinders for the JWST. The HST was launched in 1990 into a low earth orbit (LEO) at an altitude of 538 km and at an inclination, or orbital tilt, of 28.5 degrees. In 2009, the KST was launched from Cape Canaveral into an orbit around the Sun. In July 2015, the KST discovered the planet Kepler-452b, which is about 1,400 light years away from Earth. Kepler-452b is the first near-Earth-sized world to be found in the habitable, or Goldilocks zone, of a star that is similar to our Sun (Figure 3). The Goldilocks zone is a region around a star where temperatures are ideal for water to pool on its surface (Sawe, 2017). The Kepler Space Telescope ran out of fuel needed for operations and was retired in 2018 after finding more than 2,600 exoplanets (Johnson, 2015). Overall, over 5500 exoplanets have been discovered by our telescope assets (NASA, 2021).
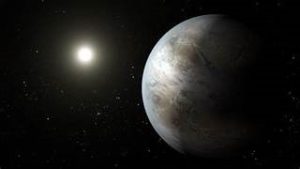
Another advantage of space systems is the free-fall environment of space. It does not just make astronauts queasy and give them chicken legs and fat faces! It has many advantages that have led to advances in materials science, food growth, and biological research. But what is meant by free-fall?
Is it zero gravity? No! It simply means there are no contact forces on the body. In the case of satellites in orbit, the satellite is falling toward the earth because of gravity, but it is continually missing the earth because of its forward speed and the earth’s curvature. We often refer to this as microgravity. You will understand more about this topic in chapter 1.
In microgravity, crystal structures tend to grow larger and with fewer impurities than they do on the surface. Shown in Figure 4 on the left is an example of protein crystals grown in microgravity. Compare them to those grown on Earth on the right (Choi, 2016).
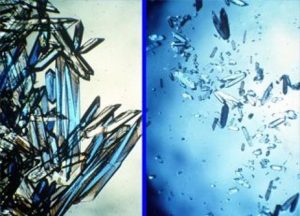
The flatworm shown in Figure 5 was originally an amputated flatworm fragment sent to the International Space Station in 2017. If you look closely, it has two heads with two separate sets of eyes, which is an interesting phenomenon that occurs due to free-fall (Fredette, Smith, Sable, & Kasap).
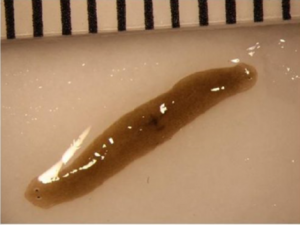
Space has a vast availability of resources. Not only do we have a practically unlimited supply of solar power, but there are also minerals and other extraterrestrial materials out there. We know the moon alone has an ample supply of ice at the poles, rich regolith, and abundant Helium-3 (He-3), which is scarce on Earth. He-3 has the highest energy to mass ratio of any substance found in nature. When combined with deuterium, or heavy hydrogen often found in Earth’s oceans, it can be used for fusion reactors. It is possible to get highly efficient and powerful engines from a He-3/deuterium combination, up to 55 times more efficient than any chemical rockets in use today (Javier, 2019).
There are any number of resources available on other planets. Just take the red planet, Mars, for example, shown in Figure 6. Its color alone indicates an abundant supply of iron. Other ores also exist on Mars including nickel, copper, titanium, platinum, palladium, and chromium.
![An image of Mars showcasing not only the highest point in the solar system, Olympus Mons, but also a canyon 4000 km [2500 miles] long called Valles Marinaris.](https://oer.pressbooks.pub/app/uploads/sites/30/2020/12/Mars-300x300.jpg)
Mars also features Olympus Mons, which is the tallest mountain on the planet, and the largest in our solar system. Valles Marineras Canyon is Mars’ version of Earth’s Grand Canyon, but a whopping 4000 km (2500 miles) long. It is large enough to stretch the distance across the United States from coast to coast. We can only imagine what resources this and other features on Mars may contain, not to mention the resources on other planets (Redd, 2017).
Space is an adventure for the human race – exciting yet challenging. It is human nature to want to explore the unknown, push the limits of what we can accomplish, do what nobody else has done before, and make new discoveries. It is a unique challenge.
I can only image the excitement the world felt when Neil Armstrong and Buzz Aldrin took their first steps on the moon. We have had plenty of discoveries throughout the years that have both intrigued and piqued man’s curiosity. One of them, aptly named the Mars Curiosity Rover, did just that (Figure 7). Early in its mission, in Gale Crater, Curiosity found chemical and mineral evidence of past habitable environments on Mars. We are learning so much about Mars’ climate and geology from the chemistry and structure of the rocks and soil Curiosity’s 10 science instruments and 17 cameras have found (Mars Science Lab, 2019).
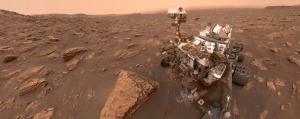
There are many, many other discoveries that have been made just in our galaxy. The Cassini mission visited Saturn and its moons beginning in 2004, New Horizons visited Pluto in 2015, and Juno explored Jupiter starting in 2016. The Dawn mission explored some of the largest asteroids in our solar system: Vesta in 2011, and Ceres in 2015. Data gathered was in both visible and infrared wavelengths, and we found that both planet-like worlds evolved very differently (Figure 8). OSIRIS-REx was the first US mission to collect a sample from an asteroid, Bennu, which was returned to earth on Sept 24, 2023. NASA is always interested in exploring other worlds to expand our understanding of the solar system (NASA Dawn, 2020).
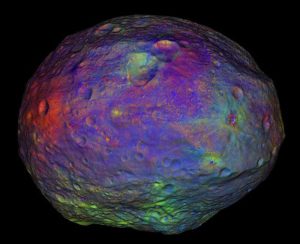
So now that you have some background on the reasons for space missions, let us discuss some types of space missions. Pause for a minute to think about some types of space missions you have heard about. We will discuss these in the next section.
For a recap of this section, see Video 3.
Video 3: Course Introduction
TYPES OF SPACE MISSIONS
Space systems are widely used in our daily lives. We rely on space systems to help us navigate around on Earth. We also use space assets for weather monitoring, television, communication, and to develop many technologies that we use every day. NASA has a website that highlights space technologies that benefit life on Earth in the form of commercial products. NASA has profiled more than 2,000 spinoffs since 1976 – there is more space in your life than you might think! (NASA, 2020).
First up is space-based communication. One set of satellites NASA uses is TDRSS, or the Tracking and Relay Data Satellite System (Figure 9). It is a network of satellites and ground stations that allow spacecraft to communicate with the ground as needed. As the mission manager for a satellite launched on the Titan IV launch vehicle, I used TDRSS to communicate closely with the ground during the launch phase. TDRSS has provided critical communication to thousands of satellites.
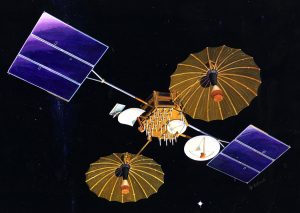
The Mobile User Objective System (MUOS) is an ultra-high frequency (UHF) satellite communication system developed for the US Navy (Figure 10). MUOS satellite radio terminal users can connect around the globe into the Global Information Grid as well as into the Defense Switching Network. MUOS provides voice, video, and mission data on a high-speed Internet protocol-based system.
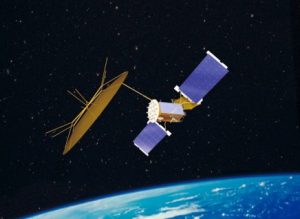
The Wideband Global Satcom System, WGS, is a US satellite constellation that provides military communication around the globe (Figure 11). The system was initiated in 2001 as a replacement for the Defense Satellite Communication System, DSCS, which operated from the 1980s into the 2000s (WGS, 2020). Although DSCS performed extremely well for many years, WGS delivers newer and greater capabilities and provides the primary communication for the Department of Defense. It operates alongside the Air Force’s Advanced Extremely High Frequency, AEHF, and the Navy’s Mobile User Objective System satellite communication systems. In fact, the US Space Force’s first launch was the AEHF-6 satellite on a United Launch Alliance Atlas V rocket in March 2020 (Blastoff!, 2020).
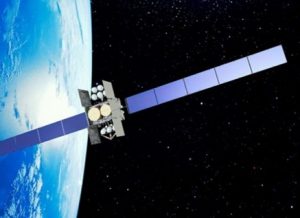
The Advanced Extremely High Frequency (AEHF) program is a constellation of communication satellites operated by the US Space Force (Figure 12). They are used to relay security communication for the US, British Armed Forces, the Canadian Armed Forces, the Netherlands Armed Forces, and the Australian Defence Force. The AEHR system is a joint service communications system that provides survivable, global, secure, protected, and jam resistant communications for high priority military ground, sea, and air assets.
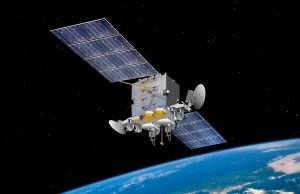
Most satellite communication systems operate from geostationary or geosynchronous orbits. GEO orbits are at an altitude of about 35,800 km (22,250 miles). Satellites at that altitude with an inclination, or orbital tilt of 0º are called geostationary. Those at an inclination other than zero are called geosynchronous. You will learn more about these orbits and why they are useful for communication systems a little later.
Remote sensing is an important type of space mission. Shown here are only unclassified satellite systems, but remote sensing is a lot of what people think of as “spy satellites” that can be used to monitor troop movement and other land-based activities. Some of the more well-known systems are the Landsat and SPOT satellites.
Landsat is a US satellite system that shows Earth from space (Figure 13). Since the first Landsat was launched in 1972, the satellite systems have collected data on forests, urban areas, and fresh water. Its data is freely available and has helped us to better understand environmental changes, manage agricultural activities, allocate water resources, and respond to natural disasters. Landsat 8, shown below, is in a near-polar orbit called sun-synchronous. It repeats its orbital pattern every sixteen days (Jenner, 2015).
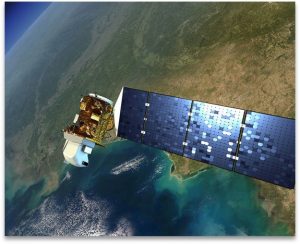
The French SPOT, Satellite pour l’Observation de la Terre, satellites have been providing high resolution optical imagery since 1986 (Figure 14). The system consists of five satellites and has revealed the surface of the Earth in enough detail that it has led to new applications in mapping, vegetation monitoring, land use and land cover, and the impacts of natural disasters. Shown here is a photo of Brisbane, Australia taken from the SPOT-6 satellite (SPOT-6, 2017).
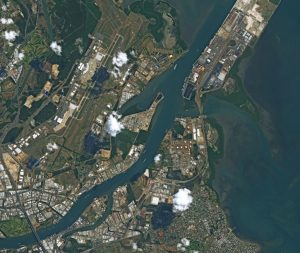
SBIRS, or the Space-Based Infrared Radar System, is a US space surveillance system that provides key capabilities in the areas of missile warning, missile defense, and defensive and offensive space characterization. It is operated by the US Space Force and consists of satellites in geostationary orbits and highly elliptical orbits. It can identify a missile’s trajectory and provide real-time information to develop an appropriate defense strategy. It has sensitive infrared sensors that enable identification of missile types and has extremely precise prediction accuracy (SBIRS, 2020).
The Sentinel-3 satellite was launched to measure ocean surface topography, ocean and land surface temperatures, and ocean and land surface colors. It supports ocean forecasting systems, environmental monitoring, and climate monitoring. It is jointly operated by the European Space Agency, ESA, and EUMETSAT, the European operational satellite agency responsible for monitoring weather, climate, and the environment (Navigation, 2020).
Aiding navigation is a useful space mission. Probably the most well-known space system on this mission is the Global Positioning System, or GPS. Not only is it used to find our way around, but it can also be used for tracking, mapping, and timing for precision agriculture, transportation, and defense purposes. But, GPS is not the only space navigation system. The Russians’ Global Navigation Satellite System, or GLONASS, uses 24 satellites to provide worldwide services. These satellites are located in medium, circular orbits at an altitude of approximately 19,100 km (14,430 miles), and at an orbital inclination, or tilt of 64.8°. Again, you will find out more about these types of orbits later in the course (Blau, 2017).
Galileo is Europe’s global navigation system. It is interoperable with GPS and GLONASS, and the complete constellation will consist of twenty-four operational satellites in medium Earth orbit at 23,222 km altitude and an inclination of 56° (What is Galileo, 2020).
We have sent numerous missions to explore our solar system and beyond. The Cassini mission to Saturn made numerous discoveries, including the extraordinary weather patterns at the north pole. The hexagon shape comes from a six-sided jet stream that spans about 30,000 km (20,000 miles) across (Figure 15). It has winds of about 322 km/hr (200 miles/hour) and has a massive, rotating storm at the center. There is no other known weather feature like this in our solar system (NASA Cassini, 2013).
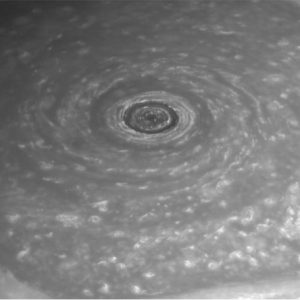
The Voyager spacecraft, launched in the 1970s, are still out there and sending back data. Voyager 1 is in interstellar space, the space between the stars, while Voyager 2 is still exploring the outer layer of the solar bubble. These missions are continuing to characterize the outer solar system environment and search for our heliopause boundary, the outer limits of the Sun’s magnetic field and outward flow of the solar wind. It is believed the Voyagers have enough electrical power and thrust fuel to keep their current science instruments on until at least 2025. Perhaps the Voyagers are destined to wander the Milky Way eternally! (Voyager, 2020).
There are many future space missions underway. Although it is impossible to predict what will happen, the human condition will continue to be improved by exploration of our solar system. A society that fails to invest in its future may have no future in the end (Sellers, 2020). For a recap of this section, see Video 4.
Video 4: Course Introduction Part 2 – Types of Space Missions Video
COURSE OVERVIEW
Now that we know some of the reasons for space systems and have reviewed some typical missions, let us take a look at what space is. Have you thought about where space begins? Well, a person who reaches 93 km (58 miles) altitude earns his or her astronaut wings. But technically, space begins at the altitude where an object will remain in orbit, at least temporarily (Figure 16).
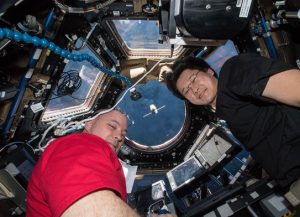
It was a 1950s engineer and physicist, Theodore von Kármán, who defined the Kármán Line, the boundary between the sciences of aeronautics and astronautics. Kármán calculated where this boundary would be. He concluded that space must begin where the atmosphere becomes too thin to support conventional airplane flight. Based on his calculations, this boundary was around 100 km (62 miles). At this altitude, a vehicle would have to travel at velocities about 8 km/s (17,900 miles per hour) in order to generate enough lift to stay aloft. At that velocity, lift would become irrelevant and the vehicle would be in orbit (Sellers & Astore).
Then, how do we get vehicles further out? There is a great program to explore this called Systems Tool Kit, or STK. As an educator or student, you can download your own free version at the website linked here (https://licensing.agi.com/stk/). This STK simulation shows one way we move satellites from smaller to larger orbits (Video 5). This maneuver is called a Hohmann transfer/combined plane change maneuver. You will learn more about maneuvering later in this course.
Video 5: Combined Plane Change
To give you a better idea of the size of relatively near space, we start at the earth, which has a diameter of 12,800 km (7850 miles). Another number you will become very familiar with in this course is the radius of the Earth, which is about an average of 6,378 km (3963 miles). Low Earth orbit, or LEO, is very close. If the Earth were the size of a peach, LEO would be about the height of the peach fuzz! It ends at an altitude of about 2,000 km (1200 miles). But where does it begin? You might guess 100 km, the Kármán Line, but we are going to use 200 km (120 miles). Below that, drag will have a major effect on the orbit. You will learn more about drag and other perturbations in this course.
Where is GEO? Geostationary orbits have inclinations equal to zero degrees, or no tilt relative to the equator. Geosynchronous orbits have inclinations other than zero degrees. All GEO orbits have periods equal to 24 hours. The orbits are at an altitude of approximately 35,780 km (22,300 miles). This allows satellites located on the equator to stay above the same position on Earth at all times. The speed of the satellite is exactly that of the earth’s rotation. To get a better sense of how far away geo orbits are, consider the earth. A geo satellite will be at an altitude of about three Earth diameters! These are excellent orbits for communication satellites.
Then, of course, further out is the moon at 384,000 km (238,600 miles), or at thirty times Earth’s diameter away. The sun is at the center of it all and is about 150 million km (93 million miles) from the earth.
The first few lectures will be on some of the fundamental laws that govern satellite orbits: Newton’s Laws, his Universal Law of Gravitation, and Kepler’s three Laws. They will help explain why satellites travel faster at periapsis, or perigee if around the earth. Perigee is the closest point in an orbit. Satellite travel slower at apoapsis, or apogee if around the earth (Figure 17).
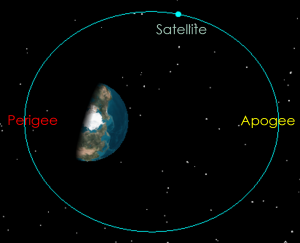
Have you ever wondered how we get something into space? Perhaps you have seen a rocket launch, live or on video. You notice how the rocket takes off straight up, but what happens after that? You will see it turn over to a nearly horizontal position. This gives it the horizontal velocity the spacecraft needs to get enough speed around the earth to stay in orbit briefly. Recall this was about 8 km/sec (17,900 miles per hour).
Next, we will study the classical orbital elements (Figure 18). These are:
a, Semimajor Axis, which describes the size of the orbit
e, Eccentricity, which describes the shape of the orbit
i, Inclination, the orbital tilt
Ω, Right Ascension of Ascending Node, or the swivel of an orbit
ω, Argument of Perigee, or the orientation of an elliptical orbit
ν, True Anomaly, which tells you where the satellite is located in its orbit
.
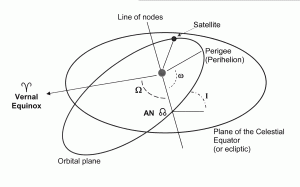
These laws, when combined, make up the two-body equation of motion for satellites. This equation and its solution will be critical to understanding orbits. Earth orbits will be referenced to the geocentric equatorial coordinate system. The video at the end if this section will help explain some of these termsWe will also look at preliminary orbit determination and ground tracks. Ground tracks are the paths a satellite makes on the surface of a planet directly below it, or the projection of a satellite’s orbit on the surface of the earth.
I had seen various types of ground tracks before but did not know until I began studying astrodynamics that you could get nearly round shaped ground tracks. These types of ground tracks are not from circular orbits! You will learn more about different types of ground tracks in this block (Figures 19 and 20).
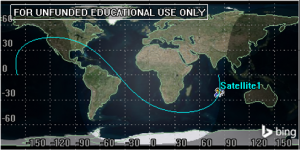
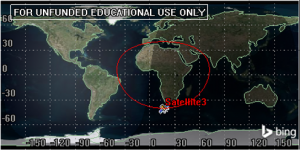
Now that you will know the basics of orbital motion and how to describe orbits, how can you predict where a satellite will be in the future so you can communicate with it? There are two types of Kepler’s problems. The first type is to find how long it takes to get from one position in space to another. This is not a difficult problem for circular orbits as the satellites in them are travelling at the same speed all the time, but what about satellites in elliptical orbits? They are constantly slowing down near apogee and speeding up near perigee. The second type is Kepler’s prediction problem. When given an initial position and time of flight, where will the satellite be in the future (Figure 21)?
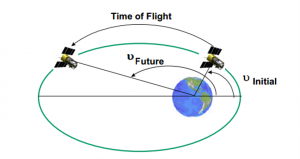
The next block will be about maneuvering satellites around in orbit. We will cover some common maneuvering techniques. First, we will consider the Hohmann transfer, which is used to change an orbit’s size (Figure 22).
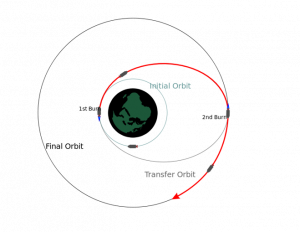
Plane changes can be used to change the tilt or the swivel of the orbit. Combined plane changes combine the two to change both the size and the tilt of an orbit (Figure 23).
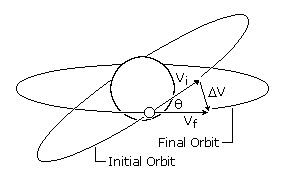
The International Space Station often receives shipments of supplies and has astronauts coming and going. Have you ever thought about how these rendezvous missions work? We will consider two types of rendezvous scenarios: co-planar and co-orbital. Co-planar rendezvous occurs when two satellites are at different altitudes and need to rendezvous. Co-orbital rendezvous occurs when you have two satellites in the same orbit. It then becomes an important issue of timing the maneuvers correctly, so the two satellites meet up together at the same time! We will also consider some more complex maneuvers (Figure 24).
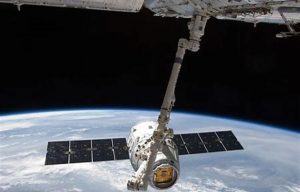
We just discussed how to predict where a satellite will be in the future. That is really only possible in an ideal world. In reality, satellite orbits are subject to disturbances, or perturbations. We will consider two main ones, geopotential and drag effects. The geopotential effect, often called the J2 effect, describes the earth’s oblateness. The earth is not perfectly symmetrical. This effect will cause a torque on an orbit, which will cause the orbit to move.
Drag will reduce the size and affect the shape of an orbit and can eventually cause satellites to de-orbit. Below is the Russian MIR space station, which reentered Earth’s atmosphere in 2001 (Figure 25). Fortunately, most of its pieces disintegrated in the atmosphere and the pieces that did not land in the ocean.
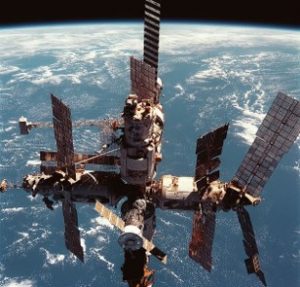
A course in astrodynamics would not be complete without at least a brief discussion of interplanetary travel. In this block, we break the multi-body interplanetary transfer problem into a series of two body problems that we will study, enabling each section to be solved for and then “patched” together. Hence, this technique is called the patched conic approach. Finally, we will take a look at gravitational assists, which are often used in interplanetary travel. For example, the Cassini mission flew by Venus twice, Earth, and Jupiter on the way to Saturn. As it passed by each planet, it got a velocity boost, enabling Cassini to reach its destination more efficiently.
If time allows, a few additional topics are recommended, which are provided in the last few lessons. Launch windows and time will give you an introduction to when and where to launch. The launch velocities lesson will show you how to determine the velocity boost needed to get there. Finally, the last two lessons will consider some more advanced astrodynamics topics. For a video overview of the book and course, see video 6.
Video 6: Course Introduction Part 3 – Course Overview
SLIDES
Course Introduction Pt 1 – Overview
Course Introduction Pt 2 – Types of Space Missions
Course Introduction Pt 3 – Course Overview
VIDEOS REFERENCES
Instructor Information
Course Introduction Pt 1 – Overview
Mice aboard the International Space Station. (2019, April 11). https://youtu.be/q7lgj3aZ8dU
Course Introduction Pt 2 – Types of Space Missions
Observing Weather From Space. (2020). https://www.e-education.psu.edu/meteo3/l5_p2.html
Ray, J. (2014, December 18). Newest GPS satellite goes active. https://spaceflightnow.com/2014/12/18/newest-gps-satellite-goes-active/
SBIRS – Spacecraft & Satellites. (2020). https://spaceflight101.com/spacecraft/sbirs/
SPOT-6 Satellite Image of Brisbane, Australia. (2017). https://www.satimagingcorp.com/gallery/spot-6/spot-6-brisbane-australia/
SPOT 7. (2020). https://earth.esa.int/eogateway/missions/spot-7
Voyager – The Interstellar Mission. (2020). https://voyager.jpl.nasa.gov/mission/interstellar-mission/
WGS – Wideband Global Satcom – Spacecraft & Satellites. (2020). https://spaceflight101.com/spacecraft/wgs-wideband-global-satcom/
What is Galileo? (2020). http://www.esa.int/Applications/Navigation/Galileo/What_is_Galileo
Wu, K. (2019, December 12). The European Space Agency Is Sending a Robot to Hug Junk Out of Space. https://www.smithsonianmag.com/smart-news/european-space-agency-sending-robot-hug-junk-out-space-180973762/
BOOK REFERENCES
Instructor Information
Doupe, C., Swenson, E., George, L, and Black, J. Finite Element Model Tuning with 3D Mode Shapes from FalconSAT-5. (2009, May). 50th AIAA/ASME/ASCE/AHS/ASC Structures, Structural Dynamics and Materials Conference.
Dunbar, B. (2012, October 15). A Long and Winding Road: Cassini Celebrates 15 Years. https://www.nasa.gov/mission_pages/cassini/whycassini/cassini20121015.html
George, Lynnane E. (2002). Active Vibration Control of a Flexible Base Manipulator. Doctoral dissertation, Georgia Institute of Technology.
George, L. E., & Kos, L. D. (1998). Interplanetary mission design handbook: Earth-to-Mars mission opportunities and Mars-to-Earth return opportunities 2009-2024. Huntsville, Ala.: National Aeronautics and Space Administration, Marshall Space Flight Center.
George, L.E. (1991). Wind Profile Data Gap Study. (ATM NO: 91-(6530-06)-10. The Aerospace Corporation.
Herbert, K. (2020). Falconsat-3. https://directory.eoportal.org/web/eoportal/satellite-missions/f/falconsat-3
Pike, J. (n.d.). Space. https://www.globalsecurity.org/space/library/news/1999/n19991220_992265.htm
Progressive Management. (2014). NASA Space Technology Report: Pogo in Rockets and Launch Vehicles.
Kepler-452B: A second earth?. The Science Explorer. (2015, September 8). http://thescienceexplorer.com/universe/kepler-452b-second-earth
NASA. (2021, March 22). Exoplanet catalog. NASA. https://exoplanets.nasa.gov/discovery/exoplanet-catalog/
USAF, Kirtland AFB. Air Force Research Laboratory’s Roll-Out Solar Array (ROSA) (af.mil)
Wilke, P., Johnson, C., Grosserode, P., & Scuilli, D. (2000, March). Whole-Spacecraft Vibration Isolation for Broadband Attenuation. https://apps.dtic.mil/dtic/tr/fulltext/u2/a451903.pdf
Course Introduction Pt 1 – Overview
Aeolus. (n.d.). http://www.esa.int/ESA_Multimedia/Images/2003/10/Aeolus
APOD: 2015 February 8 – Carina Nebula Dust Pillar. (n.d.). https://apod.nasa.gov/apod/ap150208.html
Choi, Charles. (2019, April 15). The First Detailed Study Of How Mice Behave In Space Reveals Strange, Coordinated Zooming. https://www.space.com/mice-adapt-to-living-in-space-video.html
Choi, C. (2016, August 17). Space May Be the Best Place to Grow Bone Formation Protein Crystals. https://www.space.com/33770-bone-protein-crystals-grow-better-in-space.html
Dvorsky, George. (2019, April 12). The First Detailed Study Of How Mice Behave In Space Reveals Strange, Coordinated Zooming. from https://www.gizmodo.com.au/2019/04/the-first-detailed-study-of-how-mice-behave-in-space-reveals-strange-coordinated-zooming/
Early U.S. rocket and space launch failures and explosion. (2007, June 28). https://youtu.be/13qeX98tAS8
Fredette, K., Smith, A., Sable, J., & Kasap, H. (n.d.). Space Worms? https://upward.issnationallab.org/space-worms/
Google. (2020). https://www.google.com/maps/@38.8935974,-104.8017887,218m/data=!3m1!1e3
History – Remembering First Landing on Moon – 20 July 1969. (2014, July 20). https://timeglassjournal.wordpress.com/2014/07/20/history-remembering-first-landing-on-moon-20-july-1969/
In Depth. (2019, July 15). https://solarsystem.nasa.gov/missions/kepler/in-depth/
James Webb Space Telescope – Webb/NASA. (2023). https://www.jwst.nasa.gov/
Johnson, M. (2015, July 25). A Spin Around An Exoplanet Most Like Earth. https://www.nasa.gov/image-feature/a-spin-around-an-exoplanet-most-like-earth
Joint Chiefs of Staff. (2023). https://www.jcs.mil/Media/Photos/igphoto/2001953026/
Mars Science Laboratory. (2019, September 07). https://mars.nasa.gov/mars-exploration/missions/mars-science-laboratory/
Military History Now. (2012, July 05). The First Air Forces – A Century of Balloons at War. https://militaryhistorynow.com/2012/07/05/early-air-power-100-years-of-balloons-at-war/
NASA Dawn. (2020, November 04). https://solarsystem.nasa.gov/missions/dawn/overview/
NASA. (2023, September 14). NASA’s Webb takes star-filled portrait of pillars of creation. NASA. https://www.nasa.gov/universe/nasas-webb-takes-star-filled-portrait-of-pillars-of-creation/
NASA Jet Propulsion Laboratory Blog. (2014, May 23). https://www.jpl.nasa.gov/blog/2014/5/vesta-360/
Redd, N. (2017, December 09). Valles Marineris: Facts About the Grand Canyon of Mars. https://www.space.com/20446-valles-marineris.html
Ten Years Aboard the International Space Station. (2010, November 02). https://launiusr.wordpress.com/2010/11/02/ten-years-aboard-the-international-space-station/
Sawe, B. (2017, December 12). What is the Goldilocks Zone? https://www.worldatlas.com/articles/what-is-the-goldilocks-zone.html
Sellers, J. J., & Astore, W. J. (2020). Understanding space: An introduction to astronautics. New York: McGraw-Hill.
StarTrek’s Monologue. (2008, January 25). https://youtu.be/S6R3MiAv9ac
Javier, Yanes. (2019, April 01). Helium-3: Lunar Gold Fever. https://www.bbvaopenmind.com/en/science/physics/helium-3-lunar-gold-fever/
Course Introduction Pt 2 – Types of Space Missions
Blau, P. (2017, September 23). Glonass Satellite Blasts Off on Soyuz to Replenish Russian Navigation Constellation. https://spaceflight101.com/soyuz-glonass-m-52-launch/
Dunbar, B. (2015, May 01). Tracking and Data Relay Satellite (TDRS) Fleet. https://www.nasa.gov/directorates/heo/scan/services/networks/tdrs_fleet
Flybys. (2019, March 20). https://solarsystem.nasa.gov/missions/cassini/mission/tour/flybys/?page=0
Jenner, L. (2015, April 01). Landsat Overview. https://www.nasa.gov/mission_pages/landsat/overview/index.html
MUOS. (2020). https://www.lockheedmartin.com/en-us/products/muos.html
NASA. (2020). https://spinoff.nasa.gov/
NASA’s Cassini Spacecraft Obtains Best Views of Saturn Hexagon. (2013, December 04). https://www.jpl.nasa.gov/news/news.php?release=2013-350
Navigation. (2020). https://sentinel.esa.int/web/sentinel/missions/sentinel-3
Observing Weather From Space. (2020). https://www.e-education.psu.edu/meteo3/l5_p2.html
Ray, J. (2014, December 18). Newest GPS satellite goes active. https://spaceflightnow.com/2014/12/18/newest-gps-satellite-goes-active/
SBIRS – Spacecraft & Satellites. (2020). https://spaceflight101.com/spacecraft/sbirs/
SPOT-6 Satellite Image of Brisbane, Australia. (2017). https://www.satimagingcorp.com/gallery/spot-6/spot-6-brisbane-australia/
SPOT 7. (2020). https://earth.esa.int/eogateway/missions/spot-7
Voyager – The Interstellar Mission. (2020). https://voyager.jpl.nasa.gov/mission/interstellar-mission/
WGS – Wideband Global Satcom – Spacecraft & Satellites. (2020). https://spaceflight101.com/spacecraft/wgs-wideband-global-satcom/
What is Galileo? (2020). http://www.esa.int/Applications/Navigation/Galileo/What_is_Galileo
Wu, K. (2019, December 12). The European Space Agency Is Sending a Robot to Hug Junk Out of Space. https://www.smithsonianmag.com/smart-news/european-space-agency-sending-robot-hug-junk-out-space-180973762/
Course Introduction Pt 3 – Course Overview
AGI, Free Trial Options. (2021). https://licensing.agi.com/stk/
Convert Keplerian Orbital Elements to a State Vector. (n.d.). https://www.mathworks.com/matlabcentral/fileexchange/35455-convert-keplerian-orbital-elements-to-a-state-vector
Deorbit of Russia’s Mir Space Station was 10 Years Ago Today. (2011, March 23). https://siliconcowboy.wordpress.com/2011/03/22/deorbit-of-russias-mir-space-station-was-10-years-ago-today/
Orbital Mechanics 101, page 1. (n.d.). http://www.abovetopsecret.com/forum/thread460766/pg1
Sellers, J. J., & Astore, W. J. (2021) Understanding space: An introduction to astronautics. New York: McGraw-Hill.
Media Attributions
- Prof. Thaddeus Lowe Making a Balloon Ascension on a Reconnoitring Expedition to Vienna, VA. © Thaddeus Lowe adapted by Library of Congress is licensed under a Public Domain license
- Pillars of creation
- Kepler planet
- Large Protein Crystals on Left Formed in Space © NASA is licensed under a Public Domain license
- Double-Headed Worm From Space © Junji Morokuma is licensed under a Public Domain license
- Mars © NOAA is licensed under a Public Domain license
- Mars Curiosity Rover © NASA is licensed under a Public Domain license
- Color Map of Vesta Depicting Various Mineral Deposits © NASA is licensed under a Public Domain license
- TDRSS-3
- MUOS-2 © Lockheed Martin is licensed under a Public Domain license
- WGS-8 © Boeing is licensed under a Public Domain license
- AEHF
- Landsat 8 © NASA is licensed under a Public Domain license
- SPOT-6 Satellite Image of Brisbane, Australia © Astrium Services is licensed under a Public Domain license
- The Eye of Saturn © NASA is licensed under a Public Domain license
- Astronauts Scott Tingle and Norishige Kanai Watch Dragon © NASA is licensed under a Public Domain license
- Elliptical Orbit With Perigee and Apogee
- Convert Keplerian Orbital Elements to a State Vector © Darin Koblick
- Regular Shaped Ground Track
- Round Shaped Ground Track
- Kepler Problems Visualized
- Orbital Hohmann Transfer © McZusatz is licensed under a CC BY-SA (Attribution ShareAlike) license
- Plane Changes © Blog Spot is licensed under a Public Domain license
- ISS-31 SpaceX Dragon Commercial Cargo Craft Is Grappled by Canadarn2 © NASA is licensed under a Public Domain license
- MIR on 12 June 1998 EDIT1 © NASA is licensed under a Public Domain license
- Slides_pix